Stepping back to leap forward in temperature measurement
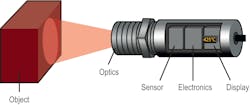
Industrial temperature sensing employs a handful of tried-and-true technologies, most commonly performed with sensors that directly or indirectly contact the target. These include thermocouples, resistance temperature detectors (RTDs) and thermistors. Each of these technologies requires physical contact between the temperature probe and the surface or product being measured. However, this approach can be problematic in certain applications.
An alternative approach involves utilizing non-contact sensing methods. Non-contact sensors provide access to applications that were previously untouchable with contact sensors. Although less widely known than standard contact technologies, infrared (IR) pyrometers have been commercially available for nearly a century. Recent advances in IR pyrometer technology make it easier than ever to cost-effectively determine temperature in a variety of critical applications without disturbing the target, and to integrate this information with automation systems.
IR radiation, blackbody principles and IR pyrometers
Traditional contact temperature measurement sensors generate a millivolt signal or vary resistance based on temperature change. These signals can be linearized and transmitted for use by host monitoring devices or automation systems. The signals are wired to input modules so that the host can use the signal for data logging, visualization and control.
Non-contact devices use IR, which is a type of electromagnetic radiation with longer wavelengths (700 nm to 1 mm) than visible light. All matter at temperatures above absolute zero emits IR radiation. According to Planck’s Law, as an object’s temperature increases, it emits more IR radiation. Using the concept of a perfect blackbody — an object which absorbs all electromagnetic radiation that falls on it — as a reference, IR detection can be used to passively determine the surface temperature of an object.
IR pyrometers (Figure 1) measure temperature without direct contact by detecting the IR radiation emitted by an object. A pyrometer is an optical device that uses a lens or mirror to focus the IR energy on a sensor. The intensity of the IR radiation is proportional to the temperature of the object. The energy focused on the sensor is converted to an electrical signal, which is then amplified and processed to calculate the temperature and transmit it to a host device.
Due to their non-contact nature and ability to measure temperature from a distance, IR pyrometers are ideal for use in industries such as manufacturing, semiconductors, chemical and food processing. Additionally, IR sensors are well suited for applications that are located near electrical interference as they are less prone to disturbances caused by nearby equipment. They rely solely on IR radiation detection, while the optical isolation within the pyrometer ensures that electromagnetic interference does not impact the temperature measurement.
IR sensors are not affected by heat conduction or convection. They are also suitable for applications with measurements between -50 and 1,600 °C in high ambient temperature, providing an option when contact thermal sensors are not viable.
It is important for designers to keep in mind that IR pyrometers only measure surface temperature — not internal temperature — which could be an issue for targets with uneven heat distribution. Furthermore, clear line of site to the target is required, and it may be necessary to adjust emissivity settings for accurate temperature results due to variations in the way certain materials emit IR radiation.
The power and perks of added proximity
By eliminating the need for the temperature sensor to physically make contact with the object or surface being measured, any interference or changes in temperature which could result from touching the object are eliminated. For instance, non-contact sensors will not distort or pull heat away from soft objects as they are being formed. Similarly, non-contact sensors can be used to accurately measure the temperature of objects carrying a current or voltage under load. An example of this is testing an operating microcontroller, where a contact sensor would remove heat from the component under test and provide inaccurate results.
Non-contact sensors are ideal for measuring the surface temperature of liquids, and monitoring can occur even as a vessel is being filled. Regardless of whether the contact is avoided for sanitary reasons — such as for a food product — or to avoid contact with hazardous materials in a chemical vat, IR pyrometers are a good choice for sensitive applications. The non-contact nature of the pyrometer avoids introducing contaminants onto the object being measured, keeping things completely sanitary. Conversely, employing non-contact sensors prevents the need for instruments to be exposed to hazardous materials.
IR temperature sensors are an excellent choice for applications where targets are in motion or cannot otherwise be physically reached. Some applications formerly used manual actions where operators needed to move a temperature sensor into position. Because non-contact sensors do not need to be applied to objects in this way, repetitive labor is reduced, and this makes them ideal choices for automating processes. Since the sensors do not have to be moved between uses, there is little wear and tear, and they often have a longer operating life than contact probes.
Rapid response
A less obvious application for a temperature sensor is high-speed counting (Figure 2). With rapid response times in the millisecond range, IR pyrometers can detect motion by measuring fluctuations in temperature within the sensor’s field of view. This makes it possible to count objects at temperature passing by, such as metal castings, glass, plastic formed bottles or even baked goods.
The IR aspect of pyrometer measurement is an added benefit where environmental factors could make implementing a photoelectric eye difficult. Whereas steam or humidity could fog a photo lens, the emitted radiation and IR detector are immune to such conditions. Similarly, in dusty environments with particulates in the air that may affect visibility, the thermal signatures in the IR spectrum remain unchanged.
Fast response time is also good for preventing damage during testing. In plastic forming, a contact temperature measurement would deform the plastic and interfere with the process. A pyrometer will measure the temperature without disturbing the surface and responsively tracks with the surface as it is formed.
Zeroing in on the target
Knowing the field of view, including the target size and distance, is crucial for selecting the right IR pyrometer lens. IR pyrometers with a close focus lens can measure a submillimeter area, a feat that is difficult to accomplish with most contact sensors. In other cases, multiple pyrometers arranged in an array or from a variety of angles can provide additional insight into the thermal characteristics of an object.
Spot size charts indicate the ratio of distance to target size. The target size is the diameter the sensor will measure at a specific distance. Pyrometers may range in spot size from 15:1 up to 300:1. This is crucial information to know during the selection and configuration process. Spot size is used to select the correct lens and focus the non-contact sensor on the surface of interest. To simplify this process, consider using pyrometers with a dual laser aiming system (Figure 3). With this type of aiming system, two lasers will intersect at the focal point where the measurement area, or spot size, is smallest. This is a considerable aid during setup.
A look below the surface — emissivity effects
The characteristics of the surface being measured affect emissivity. Emissivity is a measure of how efficiently an object emits IR radiation compared to a blackbody at the same temperature. Emissivity values range between 0 and 1, where 0 represents perfect reflection (no emission) and 1 indicates an ideal blackbody, emitting maximum radiation at a given temperature. For example, a highly-polished, shiny surface will have a lower emissivity while dark, matte surfaces exhibit a high emissivity.
Generally, surfaces with a higher emissivity will yield the most accurate temperature results when using an IR pyrometer. Most applications will provide accurate temperature results with a sensor that operates in the spectral wavelength range of 8-14 mm. For metal and metallic surfaces, sensors that operate between 1.6-2.3 mm wavelengths are recommended. Some IR pyrometers are equipped with adjustable switches to set the emissivity value. Depending on the specific pyrometer, emissivity settings may be configurable via software or mobile applications.
Despite the sensor adjustability, certain surfaces may benefit from applying coatings that increase emissivity, usually in the form of matte black tape or paints, to increase temperature accuracy. Although an unimpeded line of sight between the sensor and the object is often preferred, some IR pyrometers can accurately determine temperature through glass.
Making a choice
With a plethora of options for measuring temperature available today, it may be difficult to know which technologies to consider. While traditional temperature sensors, such as thermocouples, RTDs and thermistors, require the sensor to physically contact the surface it is measuring, non-contact IR pyrometers accurately detect temperature at a distance. Many industrial applications can benefit from non-contact temperature sensing, particularly those in hazardous environments or scenarios where contact with the sensor will have negative effects on the process or measurement. By understanding the basics of modern temperature sensing technologies, designers can confidently specify a sensor that is ideal for any application.
Bill Sonnenthal is a technical marketing engineer at AutomationDirect. He has worked at AutomationDirect since 2009 in technical and marketing roles, and he holds a BSEE degree from the Georgia Institute of Technology. Before joining the company, Sonnenthal spent 15 years designing, programming, and commissioning control systems in the newspaper and printing industry.
Billy Sonnenthal | Technical marketing engineer at AutomationDirect
Bill Sonnenthal is a technical marketing engineer at AutomationDirect. He has worked at AutomationDirect since 2009 in technical and marketing roles, and he holds a BSEE degree from the Georgia Institute of Technology. Before joining the company, Sonnenthal spent 15 years designing, programming, and commissioning control systems in the newspaper and printing industry.